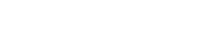
Quantum Energy Teleportation is Real!
Season 11 Episode 3 | 16m 58sVideo has Closed Captions
Quantum energy teleportation may be as close as we get to transporter beams. But how close is that?
The vacuum of space is a chaotic sea of quantum fluctuations. Some have said that this vacuum energy can be harvested to build our future starship engines. It can't. But it is technically possible to move real energy through the quantum vacuum without it passing through intervening space. Quantum energy teleportation may be as close as we get to transporter beams. But how close is that?
Problems with Closed Captions? Closed Captioning Feedback
Problems with Closed Captions? Closed Captioning Feedback
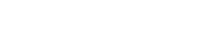
Quantum Energy Teleportation is Real!
Season 11 Episode 3 | 16m 58sVideo has Closed Captions
The vacuum of space is a chaotic sea of quantum fluctuations. Some have said that this vacuum energy can be harvested to build our future starship engines. It can't. But it is technically possible to move real energy through the quantum vacuum without it passing through intervening space. Quantum energy teleportation may be as close as we get to transporter beams. But how close is that?
Problems with Closed Captions? Closed Captioning Feedback
How to Watch PBS Space Time
PBS Space Time is available to stream on pbs.org and the free PBS App, available on iPhone, Apple TV, Android TV, Android smartphones, Amazon Fire TV, Amazon Fire Tablet, Roku, Samsung Smart TV, and Vizio.
Providing Support for PBS.org
Learn Moreabout PBS online sponsorshipThe vacuum of space is a chaotic sea of quantum fluctuations.
Some have said that this vacuum energy can be harvested to build our future starship engines, or manipulated to build warp drives.
It can't.
But it is technically possible to move real energy through the quantum vacuum without it passing through intervening space.
Quantum energy teleportation may be as close as we get to transporter beams.
But how close is that?
The laws of physics are pretty clear about a few things.
Energy can’t be created out of nothing.
Nor can energy or matter or even information travel faster than light.
The universe is very strict about enforcing these rules, but it also seems pretty cool about us exploiting loopholes.
For example, it turns out it's OK to draw energy from the vacuum of space as long as we put energy into the vacuum somewhere else.
And it’s also fine for that energy to transfer between two distant places without traveling the intervening space as long as something travels the same distance in its stead.
The protocol which makes this possible goes by the name Quantum Energy Teleportation, or QET for short, and scientists have confirmed this bizarre effect in experiments.
But before we get to that we have some theory to learn.
We have to learn about quantum entanglement, quantum teleportation, and the quantum vacuum before we can do quantum energy teleportation.
This’ll just take a few minutes with some help from favourite theoretical physicists, or should I say hypothetical physicists, Alice and Bob.
We start in Bob’s lab where he’s made a pair of entangled qubits, A and B, also known as a Bell pair.
In this case the quantum information in the qubits takes the form of the direction of the quantum spin—up or down.
For this Bell pair, the spin for each particle is undefined—it’s a quantum superposition of both up and down.
The only thing that’s really known about the spins of A and B are that they are opposite.
So Alice stops by to pick up qubit A and then goes back to her lab and measures its spin.
Let’s say she measures up.
Because this is an entangled pair, qubit B has no choice but to take on the value of down.
In other words, the wavefunction of the Bell pair collapses from up-down AND down-up to just up-down.
Alice calls Bob and tells him that he has a down qubit and he confirms with a measurement.
That’s a cute trick, but it would work equally well if the spin of the particles were fixed from the beginning.
This gets more interesting if Alice chooses different directions for her measurement—for example, measuring left-right will ensure a left or right spin, and Bob’s will still be opposite but on a different axis.
This is a real superluminal or non-local influence, and in general this sort of experiment is called a Bell test.
We’ve covered it before.
Although there are correlations between Alice’s choice of measurement axis and Bob’s readouts, it’s still not possible to send information faster than light this way.
Those correlations can only be seen when Alice communicates to Bob her measurement outputs or axis choices.
Still, it’s weird—spookily weird according to Einstein.
There are ways to make entanglement weirder however.
For example, Alice could take her half of the Bell pair and have it interact with a new, independent qubit in a way that causes Bob’s qubit to take on the quantum state of that new qubit, meanwhile destroying the version held by Alice.
It’s as though the quantum state of the new qubit is teleported.
But again, Bob can only access this quantum state after receiving classical information about the interaction from Alice.
This is “regular” quantum teleportation and it’s been achieved multiple times in experiments, with transfer of quantum information achieved over hundreds of kilometers, and even between Earth’s surface and a satellite.
Moving information around is nice, and actually useful for things like quantum computers and the quantum internet.
But what about moving actual stuff?
We’re a long way off teleporting actual matter with transporter beams, but matter is made of energy, so let’s start by trying to move energy around.
To do that we’ll need to expand the thought experiment a bit.
This time we’ll give Alice and Bob 10 Bell pairs.
If Alice measures the spin of all 10, she’ll know the spin of all 10 of Bob’s, assuming he uses the same up-down measurement direction.
Let’s add one more feature.
We’ll say the qubits are all suspended in a magnetic field so that the spin up state is slightly lower energy than the spin down state.
In the process of measuring the spin, Bob needs to either add energy into the system for the low energy spin-up states or extracts the same amount of energy for the high-energy spin down states.
Because he doesn’t know which will be up and which down, if he measures all 10 he’ll end up with a net zero energy.
But what if Alice does her measurements first, and is able to tell Bob exactly which qubits are up and which are down?
Then Bob can safely measure only the qubits that will allow him to extract energy from the system.
Alice will always have spent more energy making the measurements than Bob can extract, so energy conservation isn’t broken.
But it certainly does feel like Alice teleported her spent energy to Bob.
This all sounds a bit impractical.
For example, before they can teleport energy they need to physically exchange prepared qubits.
That’s where the quantum vacuum will finally come back into it.
As a reminder, the vacuum in quantum mechanics is not the passive nothingness of classical physics.
Every point in space is permeated by quantum fields, whose excitations give us the particles and forces.
The lowest energy state of these fields is typically zero, which means no particles—a perfect vacuum.
But due to the Heisenberg uncertainty principle, quantum systems can’t take on perfectly defined values.
So the zero energy state is only approximately zero.
It’s zero on average, but when you make a measurement at a given spot it might be a bit larger or smaller than zero, and the fluctuations witnessed by an individual series of measurements seem completely random.
Let’s try an analogy.
Imagine a pond.
There’s a very light rain, so that at any time the surface of the pond is a pattern of overlapping ripples.
The height of the ripple at any one point is subtly correlated with the entire ripple pattern.
You add a device to track the ripple height at one point.
On its own, it seems to fluctuate randomly.
But measuring at two different spots you’ll see correlations due to the fact that you’re measuring correlated ripples.
In principle, you could even extract energy from random-seeming up-down motion by using the knowledge you gain about the global ripple pattern.
Not only that, but your measurement itself causes ripples, which influences the measurements you make elsewhere on the pond.
The quantum vacuum is a bit like the surface of our pond, but now all oscillations at all frequencies—both positive and negative—are simultaneously present but balanced in such a way that the pond—the vacuum—looks still or empty.
But when you look close you can’t help but interact with the vacuum, and suddenly the wild fluctuations can become apparent.
And just like the pond surface, fluctuations at one point are correlated with all other points.
Each point is entangled with every other point in the true quantum sense.
So, while measurements of the quantum vacuum at any one point yield random energies that average to zero, those same quantum fluctuations are correlated with the fluctuations measured at any other spot, even if the spots are separated by large distances.
So the idea of quantum energy teleportation is that this quantum entanglement can be used to extract energy from one location based on measurements made at a completely different location.
It’s the same idea as our energy extraction from particle spins, except this time we don’t pre-prepare entangled pairs, instead we use the preexisting entanglement in the vacuum itself.
The process of extracting entanglement from the quantum vacuum is called entanglement harvesting.
This is an interesting topic all on its own—it suggests that two measurement devices can become entangled just by measuring spatially separated but entangled regions of the quantum vacuum.
This entanglement should be able to be used as a resource for teleportation-like protocols.
And so in 2008, physicist Masahiro Hotta proposed the protocol of Quantum Energy Teleportation.
Here’s a crude idea of how it works.
Let’s say Bob now wants to extract free energy from the quantum vacuum.
Because he’s actually studied physics, he knows that this is impossible on its own.
So, once again, Bob calls Alice.
This time Alice doesn’t even have to travel to Bob to collect an entangled particle.
The quantum vacuum at her location is already entangled with the vacuum at Bob.
She can make a measurement and send the results of that measurement to Bob by classical channels and Bob can use that information to extract real energy.
Let’s look at what the quantum fields are actually doing in the QET protocol.
As I mentioned earlier, we can think of the quantum vacuum as being made up of every possible frequency mode, which together cancel each other out to yield the apparent emptiness—zero average energy—observed on large scales.
But this balance can be disturbed.
It’s disturbed by black holes to produce positive energy outside the black hole and negative within—that’s Hawking radiation, and it’s disturbed by the measurements made by Alice and Bob.
Alice’s measurement yields a positive energy, and that means she has injected energy into the vacuum.
She disturbed the vacuum so the underlying vibrational modes no longer cancel, but rather yield an excited state.
Bob then uses the information from Alice’s measur 1ement to tune his own measurement, which ensures he sees the vibrational modes that were depleted to power Alice’s excitation.
Bob sees a negative energy density in the vacuum, and finds that the missing energy is now in his measuring device.
Alice needs to input at least as much energy as Bob extracts.
That’s why this protocol feels like energy teleportation—energy injected into the quantum vacuum at one location is extracted at a different location without it ever taking the form of real particles.
It doesn't violate energy conservation.
It also doesn't violates relativity.
Because the measurement information Alice sends to Bob still has to travel by classical channels.
This idea of extracting energy from a seemingly random, chaotic system by using detailed information about the system is not new.
It’s the same idea as Maxwell’s demon.
In this thought experiment, we start with a gas at a uniform temperature.
Energy can’t be extracted from it because it’s already in equilibrium.
But we introduce an agent—Maxwell’s demon—who carefully monitors the trajectories of particles and sorts the particles according to temperature.
This produces a temperature gradient, so work can now be extracted from the gas.
It seems like we got energy out of thin air, but really the demon had to expend energy to do the computations to sort the gas—and expends at least as much energy as was extracted.
Just like Alice had to expend energy to “sort” the quantum vacuum for Bob.
The difference with quantum energy teleportation is it’s not possible to directly monitor the local vacuum and then extract energy from it—that local activity puts more energy into the vacuum than can be extracted.
That’s what’s cool about quantum energy teleportation—it’s a way to “Maxwell’s demon” the vacuum itself from a remote location so it can be harvested.
So, is quantum energy teleportation even possible?
The answer seems to be yes, although not yet via the vacuum.
It was first demonstrated in 2022 by a team of physicists primarily based out of the Institute for Quantum Computing (IQC) in Waterloo Canada.
They used entangled qubits in the form of the quantum spin of carbon atoms in a molecule of transcrotonic acid—more commonly used to create paints and glue.
The qubits were stabilized and manipulated using nuclear magnetic resonance—the same physical phenomena which allows MRI machines to work.
A pair of entangled qubits in their lowest energy states were used as proxies for the quantum vacuum and a third qubit to mediate the measurement.
The team found that the correct measurement process enabled energy to be deposited in one qubit and then extracted from its entangled partner despite the latter being in the lowest energy state, so in principle having no energy to give.
This energy transfer occurred much more quickly than it would normally take energy to travel naturally in this system.
This successful demonstration of quantum energy teleportation was quickly followed up by an independent experiment in 2023 using IBM’s superconducting quantum computer, confirming that QET seems to be possible in realistic lab settings.
The amount of energy transferred in these experiments is very small.
The same will be true if we ever manage to do this via vacuum entanglement.
The latter will also have a very limited range.
But this could still be useful in quantum computers and various nanodevices.
But beyond moving energy around, QET experiments might be even more valuable for understanding the nature of entanglement and the quantum vacuum.
Remember that QET in the quantum vacuum leads to a negative energy density.
This is the type of energy density required in general relativity to produce exotic effects like wormholes and warp drives.
We’ve known for some time that negative energy densities are possible, for example in the Casimir effect where certain vibrational modes are excluded from the space between parallel conducting plates.
But the Casimir effect requires the presence of these plates, which more than cancel out the effect of the negative energy between them.
But the cool thing about the QET negative energy density is that it can be generated remotely, which in principle may mean that its effect on spacetime curvature can be studied.
And almost certainly will prove to be useless for faster than light motion or the inevitable capacity for time travel that results from FTL.
The universe seems to be generous only with loopholes that do NOT violate relativity this way.
But quantum energy teleportation could give us a very new understanding of the connection between negative energy, vacuum entanglement, and the curvature and even fabric of spacetime.
Support for PBS provided by: